Cell Junctions in Tissue Morphogenesis
The asymmetric distribution of membrane proteins along the apical to basal axis of a simple epithelial cell ensures that epithelial barrier and transport functions are properly regulated. However, apico-basal polarity means something different in a multi-layered epithelium such as the epidermis (Fig. 1). This tissue provides essential protection against water loss, mechanical stress, and other environmental insults. These functions require that architectural features be polarized along the entire apical to basal axis (i.e. superficial to deep layers) of the stratified epithelium, not just within an individual cell.
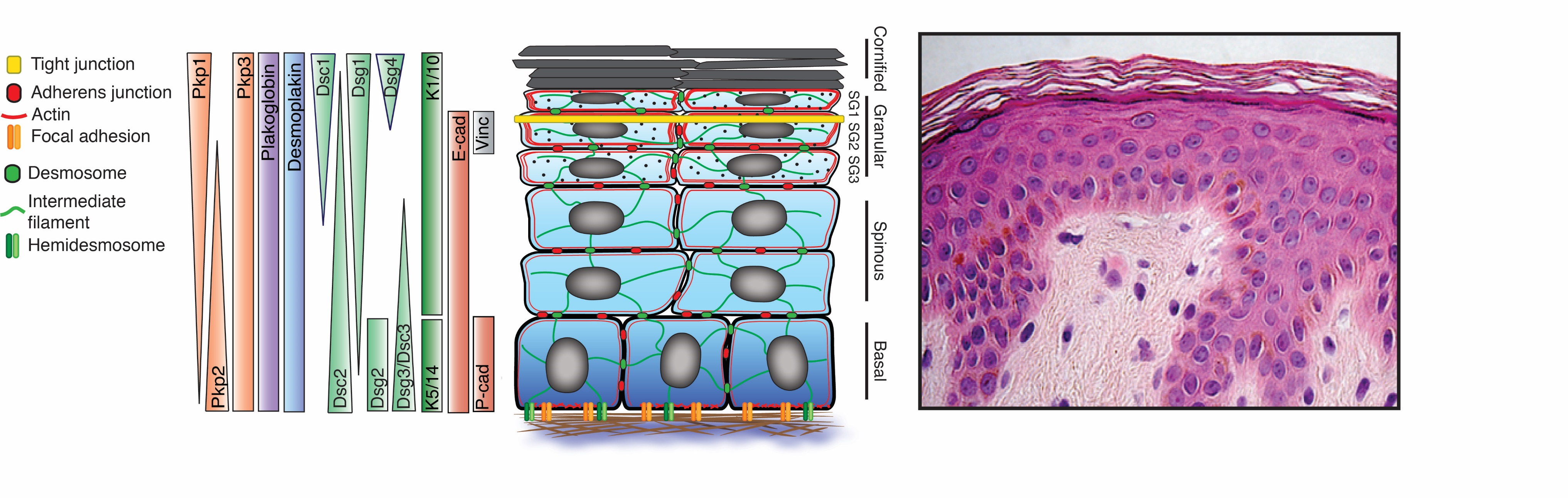
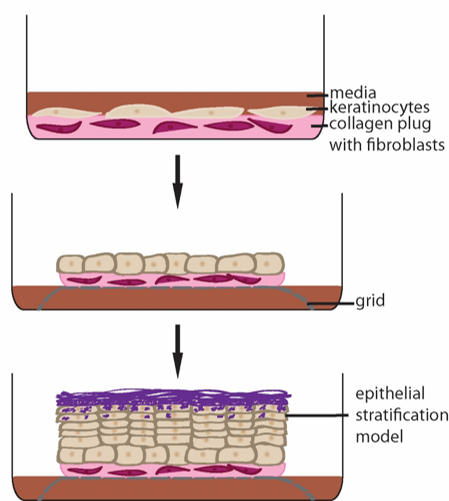
Canonical polarity proteins such as Par3/5 and aPKC have been established to play important roles in the epidermis, but contributions of other highly patterned membrane proteins to tissue polarity are poorly understood. Among the most highly polarized molecules in the epidermis are the desmosomal cadherins (Figure 1). The polarized distribution of these essential cell-cell adhesion molecules is exemplified by the reciprocal pattern of the epidermal desmogleins (Dsgs) 1 and 3: Dsg3 is concentrated in basal proliferating layers, whereas Dsg1, is expressed as cells move out of the proliferating basal layer of the epidermis and becomes progressively concentrated in suprabasal layers. Our hypothesis is that desmosomal cadherins dictate biochemical and structural information in an apico-basally polarized manner to establish a protective but dynamic barrier capable of sensing and responding to diverse mechanical and chemical stimuli. Our lab is collaborating with the lab of of Dr. Carien Niessen at CECAD, University of Cologne, to address how desmosomal and classic cadherin complexes cooperate with other polarity cues to drive morphogenesis and barrier formation in the epidermis (see Rubsam, Broussard, et al. 2017. Cold Spring Harbor Perspect. Biol.).
One of our goals is to determine how the desmosomal cadherins become reciprocally patterned in the epidermis. Towards this end, we collaborate with investigators in the Center for Advanced Microscopy using state-of-the-art optical imaging techniques to track fluorescently-tagged desmosome molecules and to determine how they segregate into distinct plasma membrane domains through microtubule- and actin-dependent mechanisms as cells differentiate. These include but are not limited to Structured Illumination Microscopy, Multi-Photon Confocal imaging and Spinning Disk Confocal imaging.
Another goal is to determine whether Dsg1 helps form the stratified tissue. We use reconstituted human epidermis to address what happens when we block Dsg1 expression (Fig. 2). These studies revealed that Dsg1 promotes the onset of expression of the biochemical program of differentiation by attenuating MAPK signaling (see “Desmosomes in Inherited Disease of Heart and Skin”). On the other hand Dsg1 promotes formation of a multi-layered tissue (stratification) by re-modeling the actin cytoskeleton which in turn transiently alters membrane tension to allow delamination of cells and formation of a second layer (Fig. 3).
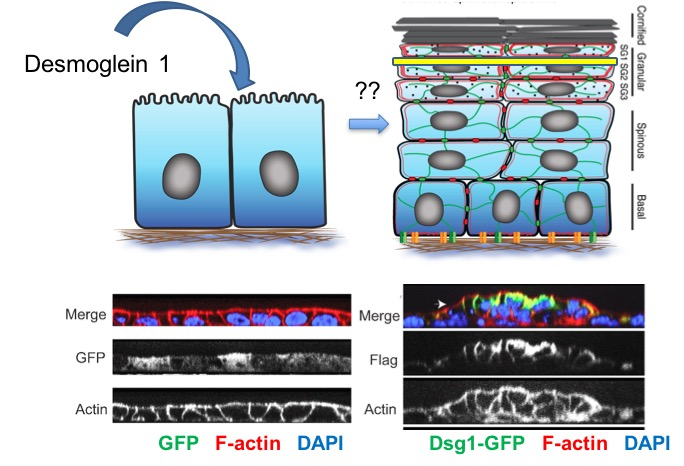
Dsg1 also controls the mechanics of the epidermis by creating a tension gradient along the apical to basal axis, with highest tension in the superficial layer where tight junctions form. Loss of Dsg1 impairs the restricted localization of tight junctions, which are also controlled by the actin cytoskeleton (Fig. 4). The cooperative roles of desmosome anchored IF and actin is being further elucidated in vitro in collaboration with Horacio Espinosa (McCormick School of Engineering) using micropillar arrays and atomic force microscopy (Fig. 5) which have shown that forces mediated by IF-DSM interactions require an intact actin cytoskeleton (Broussard, et al., 2017. Mol. Biol. Cell. 28:3156.).
Together, studies support the idea that Dsg1, which from an evolutionary perspective is a “recent” cadherin, helps both to promote formation of a multi-layered tissue and the elaboration of new cytoskeletal and cell envelope components to form a barrier that protects from stresses of terrestrial life.
Future Objectives: Our future objectives are to determine the machinery responsible for patterning desmosomes in the epidermis and how they function with chemical and mechanical signaling pathways and polarity cues to drive stratification, morphogenesis and barrier formation. One goal is to determine whether IF-desmosome connections are required for Dsg1 to drive delamination, and using a combination of genetic, biophysical and engineering tools to elucidate mechanical signaling machinery that couples to desmosomes. To determine whether findings in human reconstituted epidermis are relevant in vivo, we generated a desmoglein 1 knockout mouse through gene editing techniques. Preliminary data support the idea that these animals have thinner living layers, and we will address the extent to which defects in delamination occur during various stages of skin development and in adult skin regeneration.
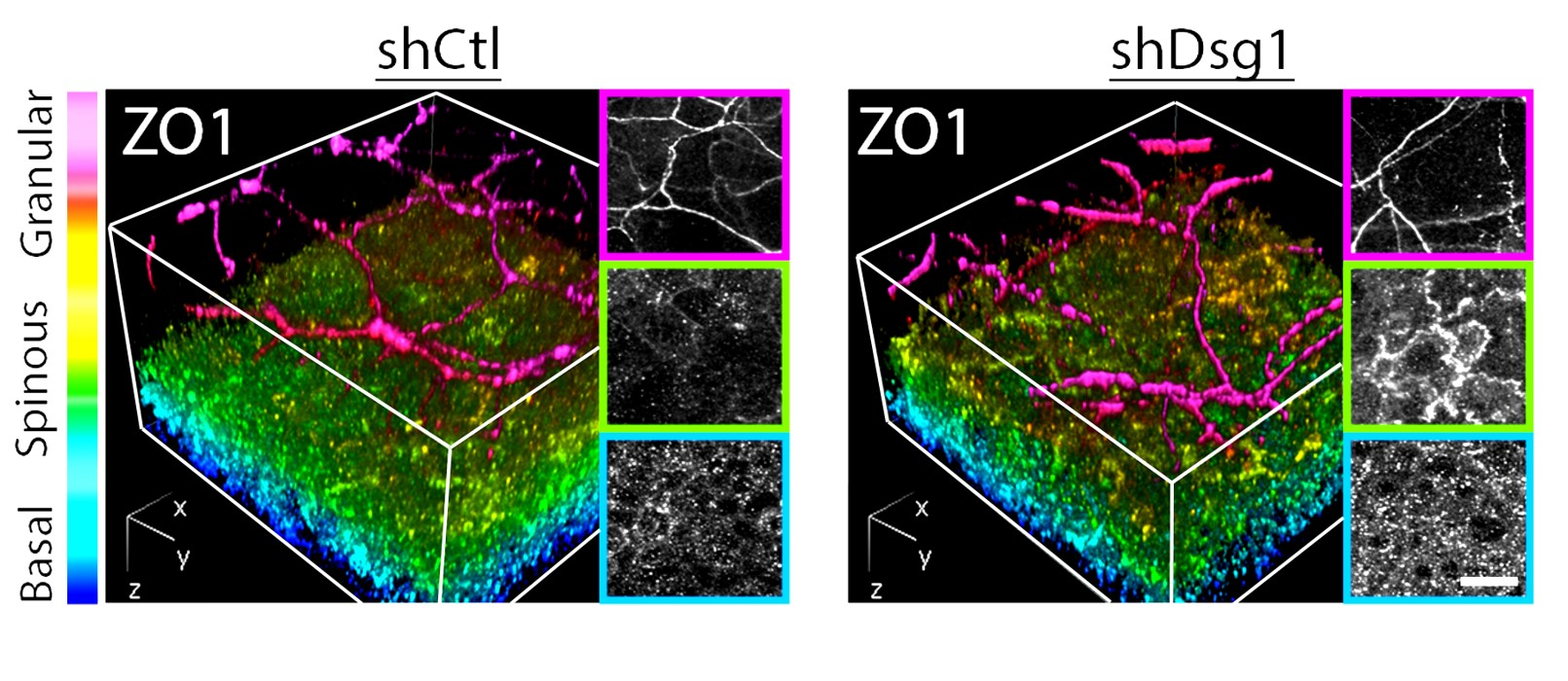
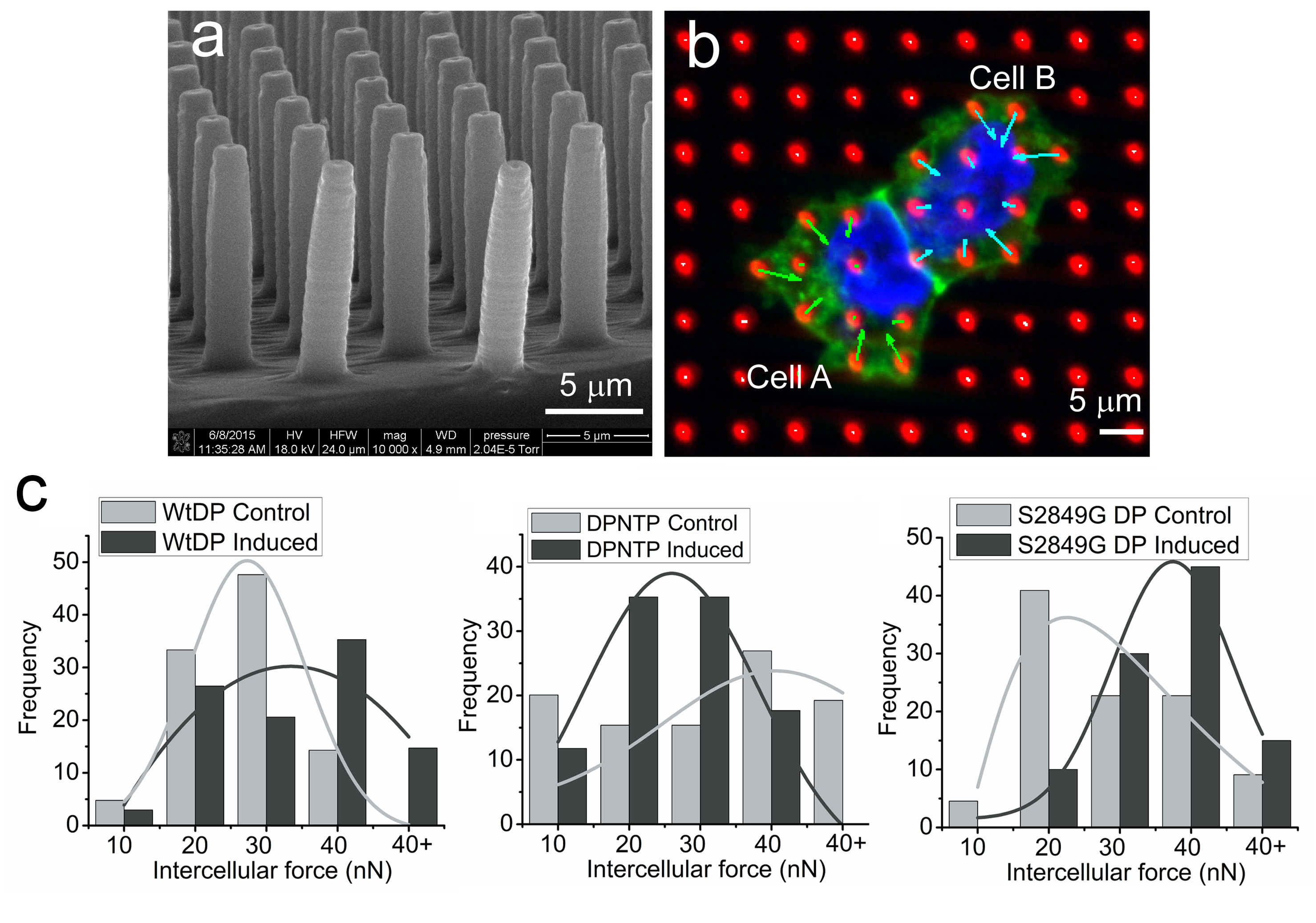