About Cytoskeletal Intermediate Filament Protein
In the cytoplasm, one, two or even more types of IF protein chains can polymerize into cytoskeletal IF of 10 nm diameter. The Types I and II IF proteins are the acidic and basic keratins and both types are required to polymerize into IF heteropolymers. In contrast, Type III proteins such as vimentin, peripherin, desmin and glial fibrillary acidic protein (GFAP) assemble into homopolymer IF. Type IV IF proteins include the classical neurofilaments composed of 3 different protein chains (NF-L, NF-M and NF-H) as well as α-internexins and nestin.
In most cells, cytoplasmic IF assemble into complex networks that course through the cytoplasm, interacting with the microtubule and actin networks. The intimate association amongst these three cytoskeletal systems is best appreciated in electron microscopic images of fibroblasts prepared for thin sectioning (see Figures 1 and 2)
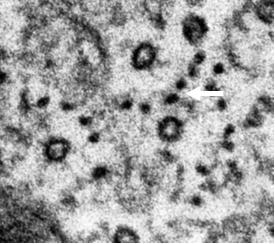
Figure 1 (left): Cross section through a long process of a hamster fibroblast. Note 25 nm microtubules surrounded by 10 nm IFs (arrow)
Toward the cell center, IF appear to attach to the nuclear envelope, and in the region of the plasma membrane, they are associated with various adhesion structures such as the desmosomes and hemidesmosomes of epithelial cells and the focal adhesions of fibroblasts.
Cytoskeletal IF play important roles in a wide range of cellular functions including the formation and maintenance of cell shape, cellular mechanical integrity, signal transduction, and the overall stability and integration of other cytoskeletal systems including microtubules and microfilaments.
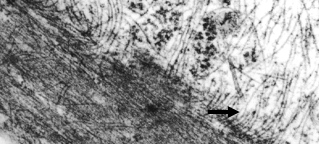
Figure 2 (right): Longitudinal section along a hamster fibroblast process showing close association between IFs (arrow) and an actin stress fiber.
Intermediate Filament Protein Structure
All IF proteins have three subdomains: a central "rod" domain that is flanked on either side by non-α-helical "head" and "tail" domains (see above image). The rod domain is mainly α-helical with a heptad repeat pattern in segments 1A and 1B forming coil 1, as well as in segments 2A and 2B constituting coil 2. These segments are joined by short, non-helical linkers (L1, L12, L2). The most conserved regions are found at the N-terminal and C-terminal ends of the rod domain, regions that play vital roles in IF assembly. There is also a highly conserved four-residue insertion near the middle of segment 2B and this so-called stutter is present in all IF.
During their assembly into 10nm filaments, individual IF protein chains interact in a hierarchical fashion. The basic building block of IF is the dimer, which self-assembles via the coiled-coil interactions of two parallel and in register IF protein chains. Two dimers associate in a staggered and antiparallel fashion to form tetramers and eight tetramers (a total of 32 monomers) combine laterally to form cylindrical structures known as unit length filaments (ULFs), with an approximate diameter of 16nm. These ULFs link up end-to-end, and through a process of compaction they are finally assembled into mature 10 nm diameter IF.
Some of Our Research on Cytoskeletal IF Proteins
Vimentin IF Are Involved in Normal Cell Motility & Cancer
During embryonic development there are interesting changes in the types of cytoplasmic IF that are expressed. Often, epithelial cells stop expressing keratin IF, and begin to express vimentin IF; when this happens, the cells break away from their partners and begin to migrate to distant locations to form new tissues, at which point they revert to keratin expression and become non-motile. The first process is often referred to at the epithelial-to-mesenchymal transition (or EMT) while the second process is referred to as the mesenchymal-to-epithelial transition (or MET). Importantly, the EMT also occurs when tumor cells in cancer patient become metastatic and migrate from the localized tumor to distant locations. So, our interest is in how vimentin changes the mechanical and motile properties of cells, and we have been able to show that introducing vimentin into an epithelial cell causesit to assume a more mesenchymal shape, and this is accompanied by a loss of desmosomal contacts, and increase in cell motililty, and a significant increase in the dynamics of focal adhesions. Likewise, silencing vimentin expression in mesenchymal cells causes them to behave more like epithelial cells.
The Contribution of IFs to Cellular & Nuclear Mechanics
Intermediate filaments differ in many ways from the two other major cytoskeletal filamentous elements. Unlike actin filaments and microtubules, IF assembly is non-polar and does not require hydrolysis of ATP or GTP. IF also have unique mechanical properties; for example when exposed to shear forces, actin filaments and microtubules rupture at very low forces whereas IF are very strong and in fact exhibit strain-stiffening in response to stress. Atomic Force Microscopy measurements show that individual intermediate filaments can be stretched to 3X their resting length before rupturing. Making use of various model systems including mouse cells from vimentin-null or lamin-null animals we are learning more about the biophysical properties of the IF. For example our collaborative work with Dr. David Weitz at Harvard, using optical tweezers for active microrheology measurements in living cells revealed that when vimentin IF are present, the cytoplasmic shear modulus is doubled. Furthermore, our AFM studies confirmed that vimentin IF can regulate both cortical and intracellular mechanics of cells. We have also used Traction Force Microscopy to characterize the traction forces and contractile state of the cells in the presence and absence of vimentin IF where we found that cell motility and contractile state are significantly compromised in the absence of vimentin IF. With respect to nuclear mechanics, both chromatin and the nuclear lamina are important contributors. By micromanipulating single isolated nuclei, we and our collaborators at Northwestern showed that chromatin governs response to small extensions while the lamins control nuclear strain-stiffening at large extensions. We are currently pursuing AFM and micro-fluidics approaches to compare normal nuclei and nuclei devoid of specific lamins or expressing disease-causing lamin mutations.
Turnover of IF Proteins
Our focus on this question is an example of how human diseases can provide important insights into fundamental cell biological questions. The rare disease known as Giant Axonal Neuropathy affects children starting around age 4 or 5, when parents notice that the child is having difficulty walking. It progresses slowly, but eventually the victim is wheelchair bound and typically dies before the second decade. The name for this disease comes from the characteristic ‘giant’ axons caused by accumulated whorls of neuronal intermediate filaments within nerve cells. Interestingly, similar aggregates of vimentin IF are seen in skin fibroblasts from these patients. A few years ago the gene that is mutated in this disease was identified, and sequence analysis suggested that the protein it encodes (named gigaxonin) was an E3 ligase adaptor involved in protein turnover via the ubiquitin-proteasomal pathway. With the availability of the sequence, we were able to introduce the gene into patient fibroblasts and show that over time the IF aggregates disappeared; and almost all the vimentin protein was eliminated as determined by immunoblotting. Moreover, over expression of gigaxonin in normal fibroblasts or nerve cells likewise resulted in loss of IF. Importantly, these insights have led to clinical trials using gene therapy to introduce functional gigaxoning genes into patients. Ongoing studies are aimed at determining the binding sites on the IF protein and how gigaxonin may be involved in initially disassembling the long IF filaments.
The Dynamic Properties of Intermediate Filaments
For many years textbooks described IF as very stable and rigid structures, only recognized for their maintenance of the mechanical stability of cells. However, the results of live cell imaging studies demonstrate the opposite. These studies have shown that IF networks are active and dynamic components of the cytoskeleton
The first studies revealing the dynamic properties of IF employed the use of fluorescence recovery after photobleaching (FRAP) (Vikstrom et al. 1992, Yoon et al. 1998). These studies revealed that there was constant exchange between subunits and polymerized IF. They also revealed that IF were not polarized with respect to subunit exchange as recovery took place at equal rates all along the length of the photobleached zones. Fast IF movements have been described in spreading fibroblasts which are enriched in non-filamentous precursors to mature IF, known as IF particles
These IF particles move bidirectionally along microtubule tracks at speeds up to ~1-2 um/min through interactions with the molecular motors, kinesin and dynein. Many of these particles assemble into short IF termed "squiggles" which in turn form the longer “filaments” found in established IF networks. Even the extensively polymerized IF networks of spread cells move, albeit more slowly, and many individual filaments appear to be constantly altering their shapes. Unlike faster particle and squiggle movements, these changes in shape, which frequently appear like propagated waves, do not require interactions with microtubules and/or microfilaments, suggesting that there are intrinsic, albeit unknown, regulatory factors responsible for some aspects of IF motility.
For more details on cytoplasmic intermediate filaments, please watch this lecture given by Dr. Goldman: